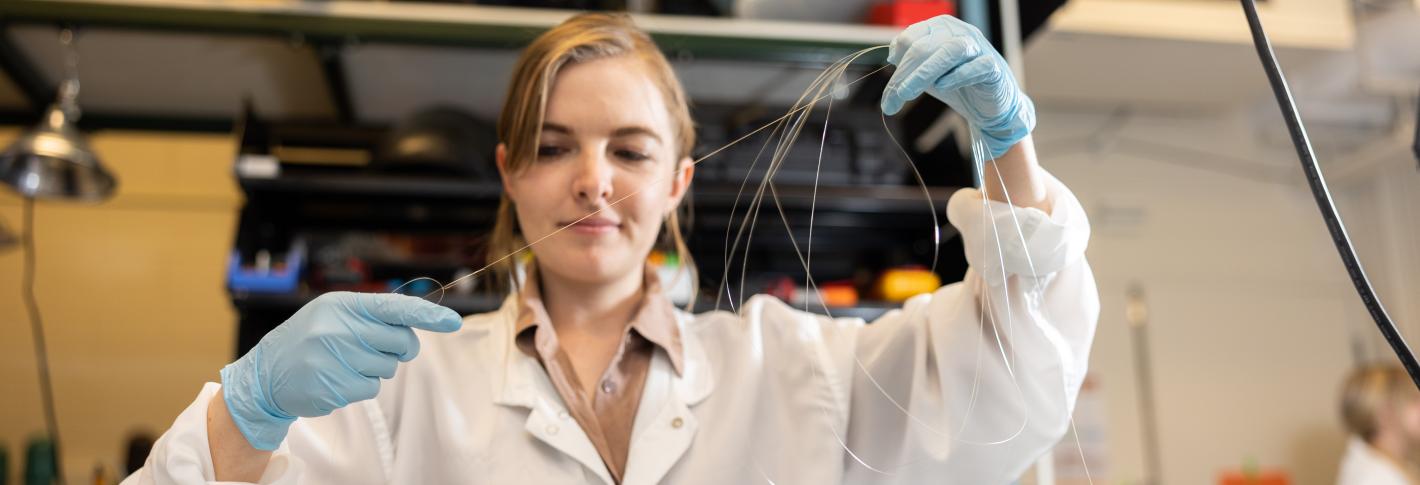
A team of researchers at MIT’s McGovern and Picower Institutes has advanced the clinical potential of a thin, flexible fiber designed to simultaneously monitor and manipulate neural activity at targeted sites in the brain. The collaborative team improved upon an earlier model of the multifunctional fiber, developed in the lab of Professor Polina Anikeeva, to explore dynamic changes to neural signaling as large animals engage in a working memory task. The results appear Oct. 6 in Science Advances.
The new device, developed by Indie Garwood, who recently received her PhD in the Harvard-MIT Program in Health Sciences and Technology, includes four microelectrodes for detecting neural activity and two microfluidic channels through which drugs can be delivered. This means scientists can deliver a drug that alters neural signaling within a particular part of the brain, then monitor the consequences for local brain activity. This technology was a collaborative effort between the groups of McGovern Institute Investigator Polina Anikeeva and Picower Institute Investigators Emery N. Brown and Earl K. Miller, who jointly supervised Garwood to develop a multifunctional neurotechnology for larger and translational animal models, which are necessary to investigate the neural circuits that underlie high-level cognitive functions. With further development and testing, similar devices might one day be deployed to diagnose or treat brain disorders in human patients.
Miller is a Picower Professor in the Department of Brain and Cognitive Sciences (BCS) and The Picower Institute. Brown is the Edward Hood Taplin Professor of Medical Engineering and Computational Neuroscience in the Picower Institute, the Institute for Medical Engineering and Science, and BCS as well as an anesthesiologist at Massachusetts General Hospital and Harvard Medical School. Anikeeva is the Matoula S. Salapatas Professor of Materials Science and Engineering, The McGovern Institute, and BCS.
The new multifunctional fiber is not the first produced by Anikeeva and her team. An earlier model engineered in their lab has already reached the neuroscience community, whose members use it to simultaneously monitor and manipulate neural activity in the brains of mice and rats. But for studies in larger animals, the existing tools for delivering drugs to the brains were rigid, bulky devices, which were both fragile and prone to causing tissue damage. A better tool was needed, both to advance cognitive neuroscience research and to set the stage for developing devices that can deliver drugs directly to the brains of patients and monitor the effects.
Above: Indie Garwood examines multifunctional fibers in the lab. Photo by Steph Stevens.
Like the devices that Anikeeva’s team designed for rodent studies, the new tool is created by first assembling a larger version of the fiber—a preform cylinder with multiple channels that is then heated and stretched until it is thin and long. As the channels narrow, microelectrodes are incorporated into to the fiber. The final step is to link the electrodes in the fiber to a connector that will relay data collected inside the brain to a unit in the lab.
The final device is long enough to access areas deep in the brain of a large animal. It is built to withstand rigorous sterilization procedures and to stay in place even in an active animal. And it integrates directly with experimental systems that cognitive neuroscientists already use in their labs. “We really wanted this to be something that we could easily hand somebody and they’re going to know how to implement it in their system,” says Garwood, who led development of the device as a graduate student in Anikeeva’s lab.
Once the new device was developed, Garwood and colleagues in the Miller and Brown labs put it to work. They used the tool to study changes in neural activity as an animal completed a task requiring working memory. The fluid channels in the fiber were used to deliver small amounts of GABA, a neurotransmitter that dampens neuronal activity, to the animal’s premotor cortex, a part of the brain that helps plan movement. At the same time, the device recorded electrical activity from individual neurons, as well as broader patterns of activity in this part of the brain. By monitoring these signals over time, the team learned how neural circuits adapted to the local inhibition they had applied. In another experiment, the team used the device to record neural activity from the putamen, a region deep in the brain involved in reward processing and motivation.
The data collected by the device was extensive and complex, tracking changes that unfolded in the brain over seconds to hours. Interpreting those data required the team to devise new methods of data analysis, which Garwood worked on closely with the Brown lab. Garwood says these methods will be shared with users of the new devices, providing “a roadmap for extracting all of these rich dynamics that you can get out of them.”
These successes, the researchers say, are an important step toward the development of tools to modulate and manipulate neuronal activity in the human brain to benefit patients. For example, they say, a multifunctional fiber might one day be used to more accurately pinpoint the origin of seizures in people with epilepsy, by testing the effects of activating or inhibiting specific brain cells.
Story by Jennifer Michalowski, courtesy of the McGovern Institute