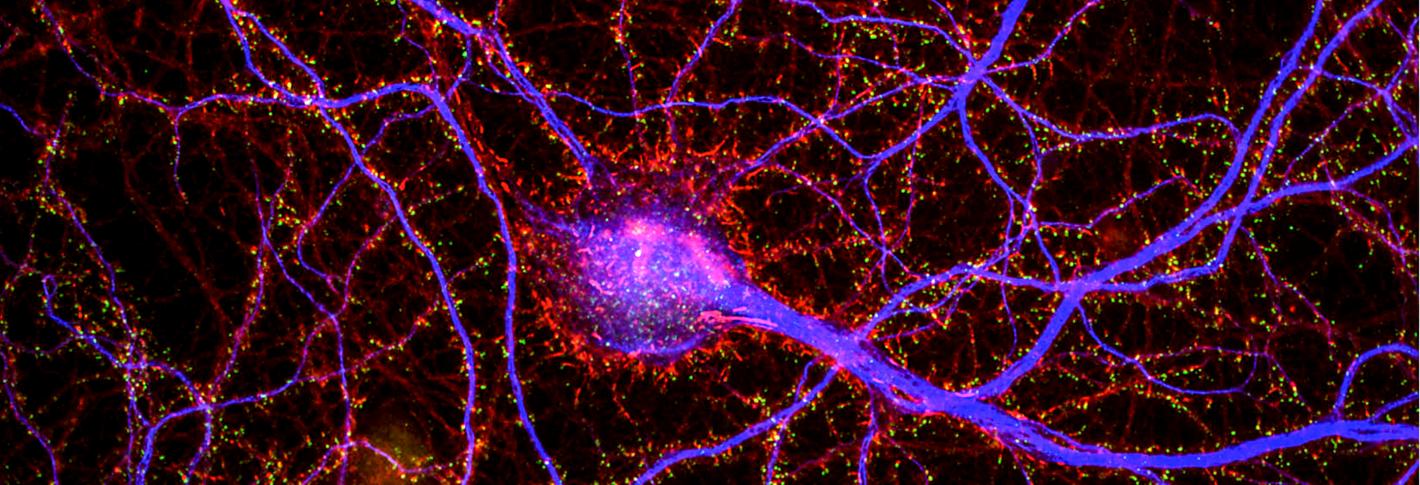
As if acknowledging the growing appreciation of their roles, not only in providing the infrastructure for brain circuit connections, but also for integrating and processing information, dendrites flamboyantly flail forth from the bodies of neurons, showing off their complex beauty. At the Picower Institute’s online fall symposium, “Dendrites: Molecules, Structure and Function” Oct. 12, neuroscientists drawn to the challenge of untangling understanding from these thickets presented many of the latest findings about how dendrites attain their intricate structure and how that, in turn, makes them versatile contributors to brain functions including perception, learning and memory.
“One of the things that people in the dendrites field have thought about for a while but is now getting broader recognition is that while neurons are computational devices, a lot of that information processing and integration happens almost immediately at the level of the dendrites,” said Elly Nedivi, William R. (1964) & Linda R. Young Professor of Neuroscience in The Picower Institute for Learning and Memory and lead organizer of the meeting. “Dendrite architecture is therefore really critical to these functions, both the anatomy and the molecular composition.”
Mindful of the ongoing need for social distancing and the Picower Institute’s intention to reduce air travel that contributes to climate change, Nedivi arranged an experimental program for the all-online symposium. One of her key goals was to ensure that even with five keynotes by senior researchers, the agenda showcased the work of junior researchers and provided fulfilling networking opportunities. Young neuroscientists delivered 10 public talks and presented in two poster sessions. At multiple private networking opportunities built into the day, senior and junior attendees also had the chance to virtually mingle.
Neuroscientists around the world responded enthusiastically to the program, which spanned a wide swath of time zones. More than 640 people from 32 countries registered to attend presentations by about 60 scientists from a dozen nations.
Insights into structure
One of the most striking features apparent in a close examination of each neuron’s dendrites is that despite their elaborate branching and extensive reach, they manage to stay out of each other’s way. Samantha Ing-Esteves, a graduate student at the University of Toronto, discussed how dendritic branches “self-avoid,” employing molecular mechanisms that trigger retraction when they come into contact with each other.
While dendrites avoid unwanted collisions, their purpose is to forge connections, or synapses, with axons (their vine-like communication partners that carry incoming signals from other neurons). In a keynote talk, Associate Professor Corette Wierenga of the University of Utrecht in the Netherlands showed that when there is a lot of activity among excitatory connections on a dendrite, it triggers a specific molecular signaling pathway to call out to nearby axons of inhibitory neurons. They then begin building new inhibitory synapses to bring in a balancing input.
In his talk, UC San Diego graduate student Daniel Heinz demonstrated how versatile such inhibitory mechanisms can be, showing that the same molecular mechanism can either produce an increase or a decrease in inhibition among a group of synapses depending on how the inciting excitatory stimulation is delivered, and where along the dendrite affected synapses reside.
The heterogeneity of which incoming signals dendrites can become tuned to process is part of what makes each neuron its own network in a sense, said Harvard University graduate student Andrew Landau. His talk described how some dendrite regions compartmentalize calcium signals to helps ensure that diversity.
A defining attribute of the synapses that line dendrites is that they are constantly changing to meet new computational needs. Scientists still have much to learn about the many ways this “plasticity” emerges, especially in live, behaving animals. Kevin Gonzalez, a Columbia University graduate student described new methods he has created to track factors affecting synaptic strength in the hippocampus as rodents perceive their movement through a space.
Sometimes plasticity along a dendrite emerges not from strengthening or weakening connections but from the creation of new ones. Graduate student Dimitra Vardalaki of the lab of MIT professor and meeting co-organizer Mark Harnett discussed her findings that new synapses can emerge, even in adults, from an abundance of “filopodia.” With stimulation, she said, these wispy structures, which she found by using a tissue expansion technology called MAP, developed by Picower Institute Associate Professor Kwanghun Chung, can grow to become synapses.
While Vardalaki looked for plasticity in adult dendrites, graduate student Jan Kirchner of the Max Planck Institute for Brain Research in Germany has developed computer models to understand how, in the earliest days of infancy, electrical signals arriving at dendrites in visual system neurons helps set up synapses to process visual information. Meanwhile in one of the symposium’s many posters, Nedivi lab postdoctoral fellow Josiah Boivin described the innovative microscopy method he uses to track how individual types of inhibitory synapses develop and mature in the visual systems of young mice.
Findings of function
Indeed dendrites enable brain functions such as sensory perception and how we make sense of it. In his keynote, Masanori Murayama, a team leader at the RIKEN Center for Brain Science in Japan, discussed his experiments showing that neurons in a brain region called the thalamus forge synapses with neurons in the cortex to regulate the response to regularly repeated, and therefore expected, touch stimulation. He used advanced imaging methods to see it in action across dendrites in a wide area of the cortex.
Professor Fritjof Helmchen of the University of Zurich in Switzerland also looked at the central role of cortical neuron dendrites in processing touch sensation. As animals learned a texture discrimination task, his team led by graduate student Gwendolin Schoenfeld is finding that different branches represent different parts of the process, such as the sensory stimulation or representation of the provided reward.
To examine how circuit connections between specific cell types in the cerebellum are architected to help the brain distinguish patterns (a key ability for learning), Harvard Medical School Assistant Professor Wei-Chung Lee, a former Nedivi lab graduate student, developed an automated system for slicing up the brain region, imaging it with a transmission electron microscope and then reconstructing the anatomy in 3D with the help of artificial intelligence. He found that the connections between granule cells and mossy fiber cells in the cerebellum appear architected with some redundancy to ensure a balance of computational robustness and processing capacity.
Several other speakers also presented ways that dendrites contribute to learning and memory. Megha Seghal, a postdoctoral scholar at UCLA, for instance, described patterns of how related contextual memories not only become represented by overlapping groups of neurons in the retrosplenial cortex, but even become linked at the level of dendrites. She has found evidence that spines responsive to two contexts that are closely associated in time appear along the same dendritic branches.
In her poster, Tomoe Ishikawa, a member of Picower Institute investigator Gloria Choi’s lab, noted that a key mechanism of memory reactivation in the hippocampus, exposure to sharp wave ripple brain waves, tends to activate spines serially along dendrites.
In episodic memory formation, neurons in a region called the lateral entorhinal cortex feeds sensory information to the hippocampus. In her talk, NYU graduate student Olesia Bilash shed new light on that conversation. To excite dendritic spikes in hippocampal neurons, LEC neurons employ VIP interneurons to create a disinhibitory network.
Sometimes when direct physical measurements haven’t yet been made, computational models can fill the gap in helping neuroscientists consider how dendrites enable learning, said keynote speaker Richard Naud, assistant professor at the University of Ottawa. He presented a model of how circuits might “back propagate” signals such as errors through multiple layers of dendritic circuitry to advance learning.
And Tejas Ramdas, a graduate student at the University of Southern California, presented his own model of what properties a neuron’s dendrites should have in order to integrate input from several others, or “pooling,” to make a summary judgement, for instance whether a particular image is detected.
For all the insights researchers revealed about the importance of dendrites, UC Berkeley Professor Marla Feller, offered something of a counterpoint: In investigating whether dendrite structures are what endow retinal cells with the ability to sense direction of moving objects, she has found that other mechanisms seem more responsible.
Dendrites may not be the root of everything, but as the symposium speakers showed, these sinuous conduits of information into neurons play substantial roles in shaping much of what the central nervous system does.