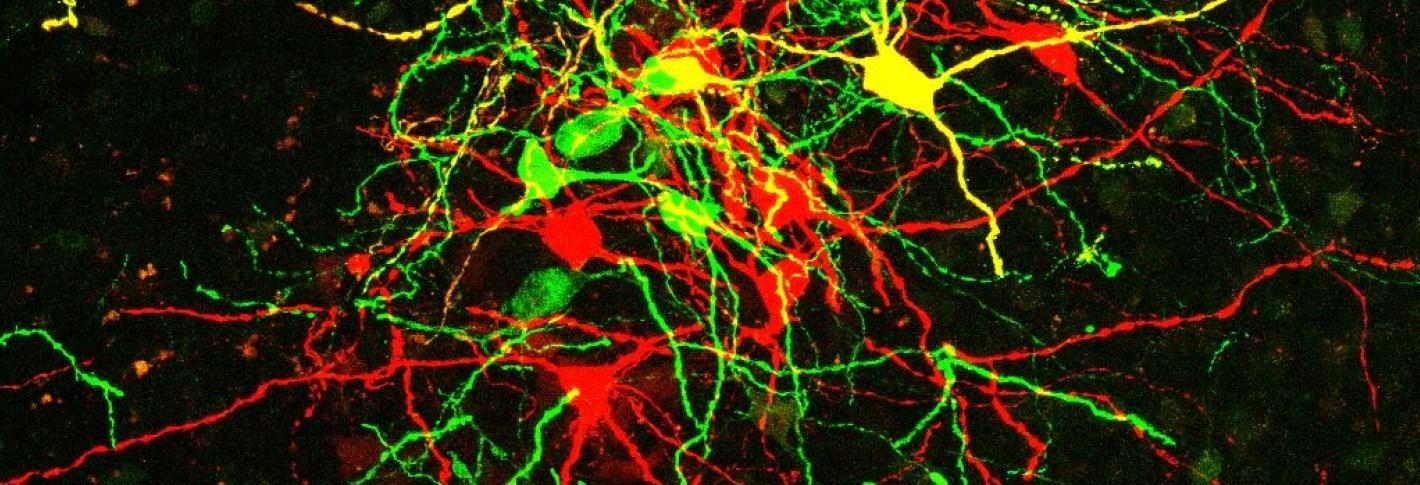
While drivers take for granted that they can approach an intersection, see a red left turn arrow and know that it is time to brake rather than turn, it is a tremendous challenge to understand how the brain accomplishes such important, everyday feats. Multiple local, regional and even brain-wide circuits and modulatory factors contribute to reliable perception, learning and applying rules, and making plans of action in response. The lab of Mriganka Sur, Newton Professor of Neuroscience, has made key discoveries that help explain how the brain reliably integrates and routes information to convert perception into appropriate goal-directed action.
Reliable perception
Some of the Sur lab’s most fundamental discoveries about brain circuits have helped to show how perception is tuned and reliable (for instance, to inform the rest of the brain). In 2005, for instance, the lab asked how the visual cortex of an animal, the part of the brain that processes incoming visual information, can consistently select for salient image features, such as the orientation of objects (e.g. that left turn arrow), even when it draws on a diversity of underlying circuits to make the computation. Through measurements and modeling, they discovered that the answer laid in how excitatory and inhibitory connections among the neurons turn out to be exquisitely balanced to fine-tune the consistency of the response.
Sur’s lab has focused in particular on understanding the role of inhibition in cortical circuits. A 2009 study, for example, showed how a particular category of inhibitory neurons (those expressing parvalbumin, or PV) helped to sharpen orientation and direction-selectivity and fine tune other attributes of vision. In 2012 the lab used a technique of selectively disabling and activating either PV or SOM inhibitory neurons to reveal that each cell type distinctly moderated visual cortex responses to stimuli. In 2021, the lab discovered how the two inhibitory neural types work together to ensure that perception can proceed reliably. PV neurons inhibit excitatory cell activity to control their gain (lest they become overwhelmed by incoming information) that can undermine the consistency excitatory representation of the same scenes by the same cells. The SOM neurons, however, inhibit PV neurons. Computer modeling showed that SOM-neuron inhibition of PV neurons kicks in when excitatory activity has become unreliable.
Taking action
Perception alone, however, only means so much. Other studies in the Sur lab have shown how it ultimately informs action. In 2016, as the scientists had mice perceive a cue and then wait to perform the appropriate action based on its training, the lab studied the simultaneous activity of three brain regions linking perception to action and discerned their contributions. They found that once the cue was shown, the visual cortex (V1) encoded its identity, the frontal motor cortex (fMC) encoded action to take, and an intermediary region, the posterior parietal cortex (PPC), represented information about both. Once that happened, only the fMC was needed to hold the course of action in memory during the wait and then execute it with the right timing. In a follow-up study in 2018 the team showed that while V1 neurons only responded to visual cues, the PPC incorporated task rules. They could even see the activity patterns of PPC neurons change when they changed the task rules.
In 2020 they looked at the interplay between two other regions in a related situation: when the same visually cued action could be reflexive or intended. For instance, a driver might turn the wheel either because the left arrow signal turns green or because of the need to avoid sudden hazard. The study reported that the anterior cingulate cortex (ACC) area of the prefrontal cortex connects with an older, deeper region called the superior colliculus (SC). The SC carries out basic commands for reactive, reflexive movement, but when planned motion is needed rather than reflex, the ACC overrides the SC via inhibitory control.
Modulatory control
Neurons become electrically active based on the signals they receive from connecting neurons (hence the metaphor of brain “circuits”) but chemicals called neuromodulators provide an important overlay across the brain that can affect how circuits function. The Sur lab has shown how certain neuromodulators affect circuits linking perception as well as learning and action.
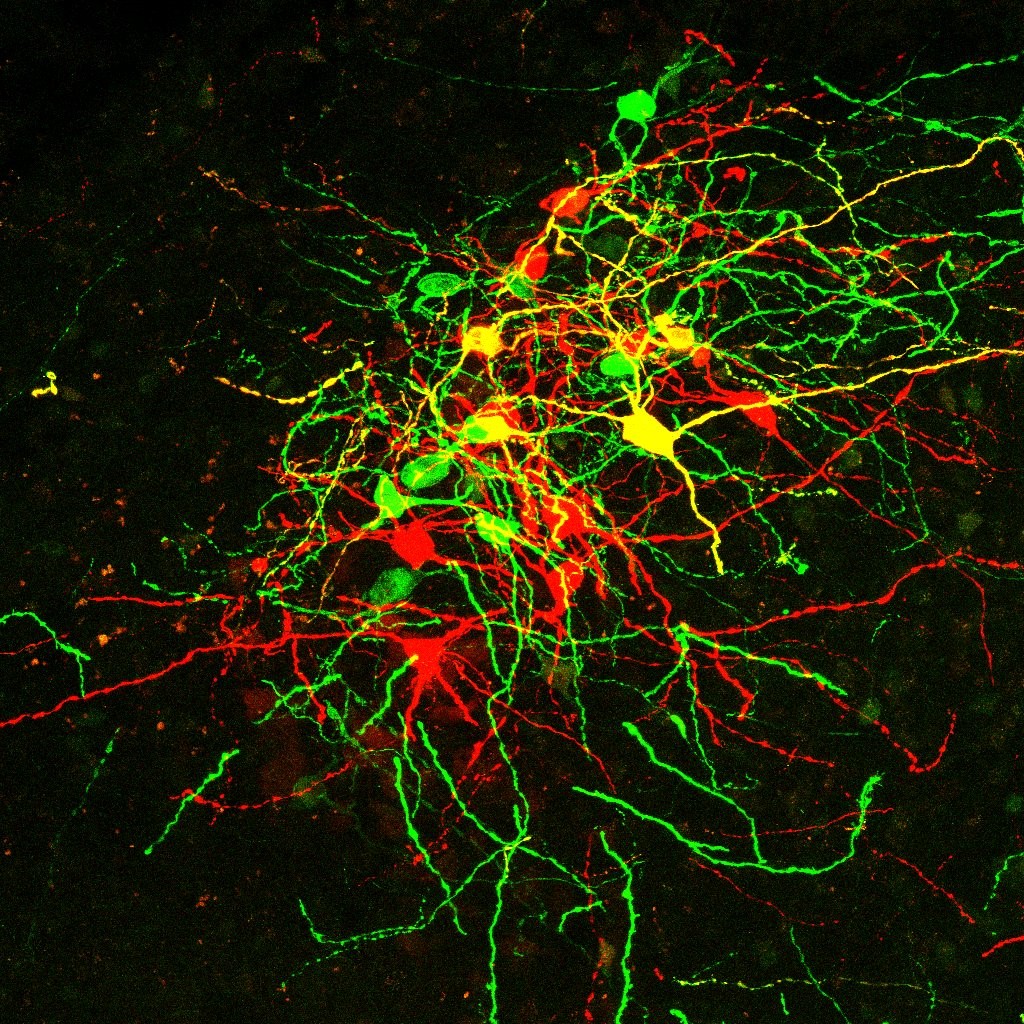
In 2015, for instance, the lab showed that the neuromodulator acetylcholine, originating from the brain’s nucleus basalis, has a crucial influence over inhibitory dynamics that affect information processing in V1, particularly because of how it influences the activity of SOM neurons. They have also discovered important ways in which the neuromodulator noradrenaline, which reaches brainwide from the locus coeruleus, controls ‘reinforcement learning’. In 2022, they demonstrated that it influences the motor cortex to promote taking sensory-cued actions that lead to reward, and that it also signals the prefrontal cortex to heed lessons brought on by surprising outcomes: for instance receiving a punishment instead of an expected reward when guessing wrong. In those ways, noradrenaline promotes both action that leads to reward, and learning when action has unintended consequences - both central components of learned behavior.